Surface Plasmon Resonance v. Quartz Crystal Microbalance
Surface Plasmon Resonance (SPR) and Quartz Crystal Microbalance (QCM) are two powerful label-free detection techniques that are receiving increasing amounts of attention for many applications, ranging from biomedical, electrochemical, and surface studies to chemical- and biosensors. An often-asked question is: Which one is better or more suitable for my applications? This note will discuss the differences between the two techniques and compare some of the key performance parameters, such as sensitivity, response time, detection limit, accuracy, and multiplexity. The goal is to provide researchers with necessary information to make a rational choice based on their applications.
Basic Principles
QCM is basically a mass loading sensor. It is sometimes also referred to as Quartz Crystal Nanobalance because nanograms of material can be “weighed” (Fig. 1). The most critical component of QCM is a quartz crystal cut in the so-called “AT orientation”, forming a keyhole-shaped disk or chip. The surfaces of the chip are patterned with electrodes, which serve two purposes: (1) drive the crystal into oscillation or resonance electrically and (2) provide a sensor surface on which molecular binding takes place. The most pronounced oscillation or resonance mode is the shear mode (displacement along the chip surface). The resonance frequency decreases upon adsorption of molecules onto the sensor surface. The frequency change (Δf) is proportional to the mass (Δm) of the adsorbed molecules per unit area, expressed in the Sauerbrey equation where CQCM is the mass sensitivity and equal to Vρ/2f2 (f is the resonance frequency, V and ρ are the shear modulus and density of quartz, respectively).
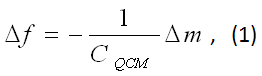
SPR is based on a different principle, but can also provide the mass of molecules adsorbed on a sensor chip– a glass slide covered with a thin layer of metal (e.g., Au). In a typical SPR setup, a light beam is directed onto the metal film through an optical prism and surface plasmons in the metal film are excited if the incident angle (resonance angle) is appropriate. The resonance angle is extremely sensitive to the polarizability and number density of the adsorbed molecules. For most biological molecules (e.g., proteins), the polarizabilities are similar, so the shift in the resonance angle is proportional to the mass of the adsorbed molecules, and is where CSPR is mass sensitivity for SPR.

The above descriptions for QCM and SPR are both simplified. However, additional considerations should be noted to better understand the differences between QCM and SPR. For QCM, molecular adsorption onto the sensor chip introduces not only a mass change but also dissipation of mechanical energy due to the internal friction of the adsorbed layer. This dissipation of energy is particularly acute when the measurement is conducted in the liquid phase (more discussion below). In the case of SPR, the conformational change of molecules bound to the surface may cause additional angular shift. These spurious contributions to the mass loading information for both QCM and SPR may be utilized to obtain additional information.
Mass Sensitivity
Sensitivity and limit of detection of a sensor are related but different parameters, although they are often mistakenly interchanged. A detailed discussion of SPR sensitivity and detection limit is given in Technical Note #102. The sensitivity of mass detection-based sensors is the mass of detected target molecules per unit area of the sensor surface for a given change of the sensor signal (frequency in QCM or angular shift in SPR). For QCM, the sensitivity is CQCM , which depends on the resonance frequency, mass density, and shear modulus of the quartz crystal. For a typical 5 MHz-QCM, CQCM can be ~ 20 ng/cm2 per Hz. A higher resonance frequency will lead to greater sensitivity of QCM. Unfortunately, a higher resonance frequency requires the already-thin crystal to be even thinner, which consequently makes the crystal become extremely fragile. For SPR, the sensitivity is CSPR , which also depends upon several parameters, such as polarizability of the target molecules, wavelength of light, and prism index of refraction (see Technical Note #102). A typical value for CSPR is ~1 ng/cm2 per mdeg.
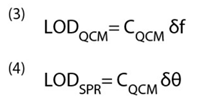
Limit of Detection
Limit of detection (LOD) describes the smallest amount of target molecules adsorbed onto the sensor that can be detected. LOD is clearly determined by the mass sensitivity, but it is also determined by how accurately one can measure the sensor output signal (frequency in QCM and angular shift in SPR). If the resolutions of the frequency and angular shift in QCM and SPR are δf and δθ, then the LODs of the two sensors are given.
For a frequency resolution of 0.1 Hz, LOD for QCM is about 2 ng/cm2 for a response time of 1 sec. This may be improved by averaging over a longer time period, say 5 sec., but this reduces the response time. It is also worth pointing out that a stability of 0.1 Hz over an entire injection peak is extremely challenging to obtain for most QCM devices (vide infra). A SPR instrument can detect 0.1 mdeg angular shift for a response time of 1 sec., which corresponds to a LOD of ~0.1 ng/cm2 , which is considerably better than that of QCM.
LOD discussed above is given in terms of detectable mass per unit area. Another useful way to define LOD is total detectable mass, denoted by LODM. The two are related by LODM=LOD × Sensor Area. A typical QCM sensor has surface area of ~1 cm2, so the total mass LOD is 2 ng. In contrast, the sensing area for SPR is determined by the size of the illumination spot*, which is often as small as 10-5 cm2. A corresponding SPR total mass LOD is 1 fg, making SPR a million times more sensitive than QCM. This means that in order for QCM to maintain a competitive sensitivity level, the sensing area must be kept considerably larger than that of SPR sensors.
It is also important to note that any external pressure (e.g., an O-ring or a gasket) applied to the QCM’s sensor surface suppresses the oscillation of the crystal, degrading its sensing performance. Thus, the entire metal-coated sensor surface (cf. Fig 1) of QCM sensors must be exposed to solution, drastically increasing its cell volume and sample consumption.
Kinetics and Binding Affinity
One of the most important tasks in studying molecular binding/interaction processes is to determine the binding kinetics, which not only allows one to extract information about the how strong a binding/interaction is, but also sheds light on the binding/interaction mechanism. To correctly extract binding kinetic information, the sample solution must be able to enter and leave the sensing area sharply and with little dispersion. This task can be readily accomplished for SPR sensors by using microfluidic flow channels and proper valving techniques. A similar fluid handling strategy cannot be easily implemented for QCM. This is because of the relatively large internal cell volume of QCM sensors (cf. Fig 1) which have to be fully exposed to sample solution.
Accuracy
The Sauerbrey equation is very accurate for gas-phase measurements. However, in the liquid environment, any non-rigidity of the molecules attached to the crystal surface will make the frequency-mass relationship deviate from the Sauerbrey equation. Any surface inhomogeneity, solvation of the film, and solution composition or viscosity change can also affect the response in QCM. These terms are collectively referred to as the “viscoelastic effect”. Although some of the effects also affect the SPR response (e.g., solution composition change and film solvation), they can be largely corrected by using a reference channel (see description below).
Multiple Channels for Background Subtraction and Multiplexity
For most biosensor applications or affinity studies, it is highly desirable to have two detection channels. In this way, one channel can be used for sample analysis and the second channel can be used as a reference or control. Referencing is best achieved by keeping everything in both channels as similar as possible, except for the testing variable. For SPR, two channels on the same sensor chip can be easily defined by pressing a patterned gasket to the sensor surface. This has the advantage of utilizing the same sensor chip and driving source (light in this case) as well as having greater surface unity in sensor surface due to their close proximity. For QCM, this method cannot be implemented as the QCM sensor would be damped, drastically degrading its performance. Referencing with QCM may be achieved through the use of two QCM chips. This method of referencing has several drawbacks: (1) the two QCM crystals must be perfectly identical to properly reference each other, (2) the delivery of sample solution to both sensors must be identical, and (3) instrumentation is considerably more complex. As a consequence, the use of referencing to correct for spurious effects such as temperature variation, electronic noise, mechanical drift, and the above mentioned viscoelastic effect is difficult to achieve. This makes maintaining the LOD, accuracy, and reproducibility of QCM measurements very challenging.
Sensor Chip Functionalization and Sample Volume
Sensor chips should to be pre-functionalized with an appropriate layer of ligand/probe or a layer of matrix to reduce non-specific adsorption. The procedures for both QCM and SPR are similar, as both utilize gold-coated surfaces. However, for in-situ or real-time pre-functionalization, QCM requires more reagents due to its larger surface area and cell volume. Moreover, uniformity of the surface functionalization becomes more difficult to achieve as the surface area increases.
Summary
Both QCM and SPR are sensitive, label-free detection methods that have found many applications in surface adsorption and bioaffinity studies. However, SPR is more sensitive, better for kinetic studies and more easily extended to multiple channels. SPR also requires far less sample volume.
Comparison between SPR and QCM
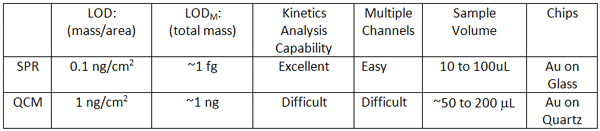
Author: Nguyen Ly | Biosensing Instrument | Published May 1, 2010
DOWNLOAD PDF
Download a PDF of Technical Note 103: Surface Plasmon Resonance v. Quartz Crystal Microbalance